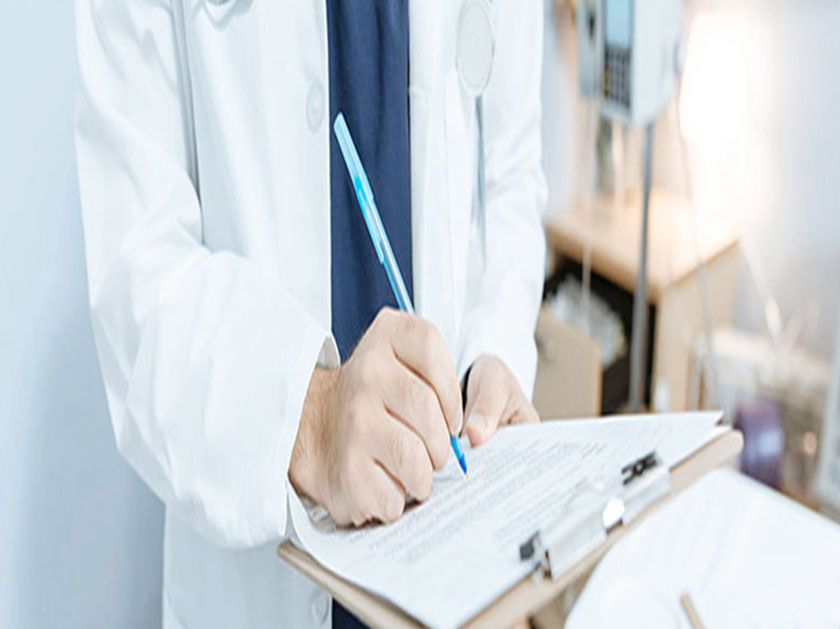
Un nuevo método portátil y sin radiación para evaluar la escoliosis: un estudio preciso y reproducible
Hui Wang1, Yunfeng Zhu1, Qiyuan Bao2, Yong Lu1, Fuhua Yan1, Lianjun Du1y Le Qin1*.
Resumen
Fondo El objetivo de este estudio era evaluar la precisión y reproducibilidad de un sistema de detección tridimensional de la columna vertebral (3D-SSS) portátil y sin radiación recientemente desarrollado para la evaluación de la escoliosis.
Métodos Un total de 145 pacientes se sometieron a imágenes de columna vertebral completa utilizando el sistema de imágenes EOS, y se recopilaron datos 3D-SSS entre febrero de 2023 y abril de 2023. Un radiólogo utilizó el software sterEOS para reconstruir la columna vertebral en 3D y obtener el ángulo de Cobb. Un radiólogo y un ortopedista midieron de forma independiente a los pacientes utilizando 3D-SSS, y el ortopedista realizó dos mediciones por paciente. El sistema de posprocesamiento 3D-SSS generó automáticamente el ángulo de Cobb.
Resultados Los ángulos de Cobb medios obtenidos mediante EOS y 3D-SSS fueron de 13,7 ± 9,9° (0,5∽45,7°) y 12,5 ± 8,6° (0,4∽40°), respectivamente. El coeficiente de correlación intraclase (CCI) para la fiabilidad entre la EOS y la 3D-SSS fue de 0,921, lo que indica una concordancia excelente. El análisis de Bland-Altman reveló un sesgo de -1,171° entre la EOS y la 3D-SSS, con sólo 10 pacientes fuera de los límites de concordancia (-8,3∽6,0°). El error cuadrático medio entre la EOS y la 3D-SSS fue de 3,2°. Se observó una fuerte correlación entre los ángulos de Cobb medidos mediante EOS y 3D-SSS (r= 0.931, P< 0.001). La curva de características operativas del receptor mostró que el rendimiento diagnóstico de la 3D-SSS para la escoliosis era de 0,953 (P< 0.001). La sensibilidad, especificidad, valor predictivo positivo y valor predictivo negativo de la 3D-SSS para el diagnóstico de escoliosis fueron 87,8%, 92,1%, 93,5% y 85,3%, respectivamente. Los CCI intraobservador e interobservador para los ángulos de Cobb derivados de la 3D-SSS fueron de 0,969 y 0,934, respectivamente, lo que demuestra una excelente reproducibilidad.
Conclusiones El 3D-SSS portátil y sin radiación midió con precisión la escoliosis y proporcionó datos altamente reproducibles. Este sistema ofrece a los médicos un método novedoso para detectar y controlar la escoliosis en pacientes jóvenes.
Palabras clave Escoliosis, Portátil, Sin radiación, Sistema de detección tridimensional de la columna vertebral, Ángulo de Cobb
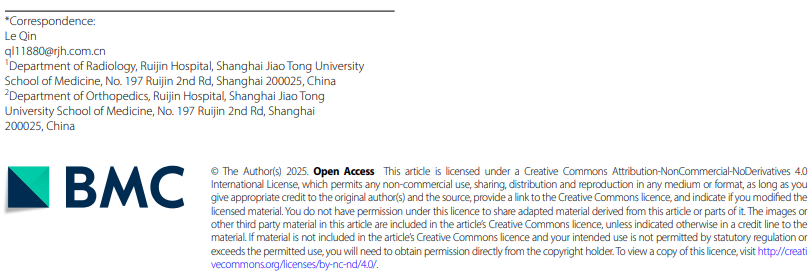
Fondo
La escoliosis es una deformidad tridimensional (3D) de la columna vertebral que afecta a los planos coronal, sagital y transversal [1]. La etiología de la escoliosis es multifactorial y en ella intervienen la genética, la biomecánica de la columna vertebral, la neurología y la bioquímica [2]. Una teoría neurológica prominente sugiere que el mal control del equilibrio postural debido a una función vestibular alterada contribuye a su patogénesis [2, 3]. Desde una perspectiva bioquímica, la baja densidad mineral ósea puede aumentar la tensión y acelerar la progresión de la curva en la escoliosis [2, 4]. El tipo más común es la escoliosis idiopática del adolescente (EIA), que se produce entre los 11 y los 18 años, con una incidencia global de 0,47-5,2% [2, 5]. La escoliosis idiopática del adulto en pacientes menores de 40 años suele evolucionar a partir de un AIS no tratado [6]. Clínicamente, el AIS grave puede dar lugar a un aspecto anormal, una caja torácica asimétrica, un deterioro de la función cardiopulmonar o una compresión de la médula espinal [7, 8]. Por lo tanto, el diagnóstico precoz y la evaluación de la gravedad del SIA son fundamentales.
Las imágenes radiológicas se utilizan habitualmente para medir el ángulo de Cobb y evaluar el plano coronal en el AIS. Históricamente, las imágenes de la columna vertebral completa se obtenían combinando múltiples radiografías, lo que provocaba distorsiones de la imagen y una importante exposición de los pacientes a la radiación. Con los avances en la tecnología de la imagen, se ha introducido el sistema de imagen EOS para evaluar las deformidades de la columna vertebral [9, 10]. La EOS ofrece ventajas como una baja dosis de radiación, la capacidad de capturar imágenes biplanares de todo el cuerpo en posición de pie y la capacidad de reconstruir imágenes en 3D [11, 12]. Sin embargo, la EOS tiene varias limitaciones, como su funcionamiento complejo, la exposición a radiaciones ionizantes, los elevados costes iniciales y el equipo inamovible, lo que la hace inadecuada para el cribado a gran escala e inconveniente para los seguimientos [13].
Para hacer frente a estos retos, se ha desarrollado un novedoso sistema de detección tridimensional de la columna vertebral (3D-SSS) portátil y sin radiación, que ofrece una alternativa a las imágenes de rayos X para evaluar los parámetros de la escoliosis. El 3D-SSS se ha diseñado para realizar exploraciones exhaustivas de la escoliosis y exámenes clínicos más rápidos. Utiliza tecnología de medición de la trayectoria en el espacio de contacto y un sensor de sistema microelectromecánico (MEMS) para medir los ángulos espaciales durante el movimiento dinámico. Combinado con la medición de la distancia de trayectoria, el sistema genera coordenadas de curvas vectoriales espaciales de la apófisis espinosa dorsal [14]. Mediante la integración de los datos de escaneado del terreno procedentes de un sensor de rueda de equilibrio del equipo y la asignación digital de estas coordenadas a un modelo estándar de columna vertebral en 3D, se puede construir un verdadero modelo digital en 3D de la columna vertebral [15, 16]. A continuación, se calculan los parámetros de la columna vertebral en el plano coronal mediante un algoritmo matemático. A pesar de su potencial, la utilidad clínica de la 3D-SSS sigue sin estar clara.
El presente estudio tenía como objetivo evaluar la precisión y reproducibilidad de las mediciones del ángulo de Cobb obtenidas mediante 3D-SSS en pacientes con sospecha de escoliosis.
Métodos
Población del estudio
Este estudio prospectivo fue aprobado por el comité de ética institucional, y todos los participantes proporcionaron su consentimiento informado firmado. Entre febrero de 2023 y abril de 2023, se inscribieron 183 individuos consecutivos según los siguientes criterios de inclusión: (1) sospecha de escoliosis; (2) edad inferior a 40 años; (3) se sometieron a imágenes de columna vertebral completa utilizando el sistema de imágenes EOS (imágenes EOS). Los criterios de exclusión fueron los siguientes (1) piel de la espalda lesionada o sensible; (2) uso de aparatos ortopédicos; (3) incapacidad para permanecer de pie durante el examen EOS; (4) presencia de fracturas vertebrales o tumores. En base a estos criterios, se excluyó a 38 pacientes, quedando un total de 145 pacientes para el análisis (Fig. 1).
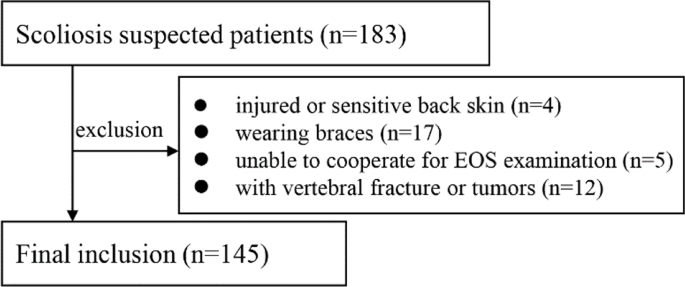
Imágenes EOS y reconstrucción 3D
Los pacientes se situaron en el centro de la zona de exploración en posición anteroposterior con las manos colocadas delante de la cabeza. Se capturaron simultáneamente dos imágenes, coronal y sagital, desde la cabeza hasta el fémur. A continuación, un radiólogo realizó de forma independiente la reconstrucción 3D utilizando el software sterEOS en modo "Fast 3D": (1) Se identificó la línea sacra oblicua y se ajustaron la posición acetabular y la inclinación pélvica en las imágenes coronales y sagitales. (2) Se determinó la curvatura de la columna vertebral (T1-L5) y se ajustó la anchura de la curvatura para que cupiera una vértebra. (3) El software generó automáticamente un modelo para cada cuerpo vertebral, que luego se ajustó manualmente para alinear la lámina terminal, la apófisis espinosa y el pedículo del arco vertebral. (4) Se identificaron manualmente las vértebras apicales, superiores e inferiores del ángulo de Cobb, que se ajustaron con precisión. Una vez finalizada la reconstrucción 3D, el programa informático calculó automáticamente el ángulo de Cobb. El AIS se definió como un ángulo de Cobb ≥ 10°. Basándose en las recomendaciones de tratamiento, los ángulos de Cobb se clasificaron en tres categorías: 10∽25° (observación), 25∽45° (corsé) y > 45° (cirugía).
Las mediciones fueron realizadas por un radiólogo con diez años de experiencia en radiología musculoesquelética utilizando el software sterEOS.
Examen con 3D-SSS
El nuevo 3D-SSS (versión: FT07W, Previsión® Spine Data Acquisition and Analysis System, Forethought [Shanghai] Medical Technology Co., Ltd, Shanghai, China) incluye un dispositivo de escaneado y un ordenador portátil de posprocesamiento. El dispositivo de escaneado cuenta con una carcasa, un interruptor, un indicador de diodo emisor de luz (LED), un rodillo sensible a la luz y cuatro rodillos de equilibrado en el exterior (Fig. 2). Internamente, contiene un módulo sensor MEMS, un codificador optoelectrónico, una placa base, una batería y un módulo Bluetooth. El análisis de los datos y las mediciones de la escoliosis se realizan mediante un software instalado en el ordenador portátil. El módulo sensor MEMS combina un giroscopio de 3 ejes, un acelerómetro de 3 ejes y un sensor geomagnético de 3 ejes, lo que permite medir en tiempo real la velocidad de rotación y la aceleración.
Cuando el dispositivo de exploración se desplaza a lo largo de la columna vertebral, los cuatro rodillos de equilibrado garantizan que el dispositivo permanezca tangente a la columna vertebral. El módulo sensor MEMS detecta los cambios de aceleración y velocidad angular en tres dimensiones (X, Y y Z). La unidad de microcontrolador (MCU) de la placa base convierte los datos de aceleración y velocidad angular en datos de cuaterniones, utilizados habitualmente en el seguimiento del movimiento en 3D. Los datos de cuaterniones se almacenan temporalmente en la memoria de acceso aleatorio de la MCU y se transmiten al software del portátil a través del módulo Bluetooth una vez finalizada la medición. El software del portátil procesa los datos de movimiento y calcula el ángulo de Cobb.
Los pasos de funcionamiento para utilizar el dispositivo de detección de escoliosis son los siguientes:
- (1)El paciente permanece de pie con las rodillas rectas y los pies colocados en posición vertical, mirando al frente con naturalidad, mientras lleva ropa fina para garantizar la precisión de las mediciones.
- (2)El operador se sitúa detrás del paciente y coloca el rodillo fotosensible del aparato a la altura de la vértebra C7, presionando ligeramente las ruedas de equilibrio contra la espalda del paciente.
- (3)El operador pulsa el botón "START" en el software del ordenador portátil y espera a que el indicador LED se ponga verde.
- (4)El operador desplaza el dispositivo a lo largo de la columna vertebral del paciente desde T1 hasta L5 (Fig. 2). Durante el movimiento, el indicador luminoso parpadea regularmente. Las ruedas de la balanza permanecen en contacto con la ropa del paciente, mientras que el operador utiliza dos dedos para asegurarse de que el rodillo optoeléctrico permanece en contacto con las apófisis espinales.
- (5)Al alcanzar el nivel L5, el aparato se mantiene inmóvil durante aproximadamente 2 s, dejando que el indicador LED deje de parpadear. El proceso de medición habrá finalizado.
- (6)El dispositivo transmite los datos de medición al software del ordenador portátil, que muestra los resultados de la prueba en 3-10 s (Fig. 3).
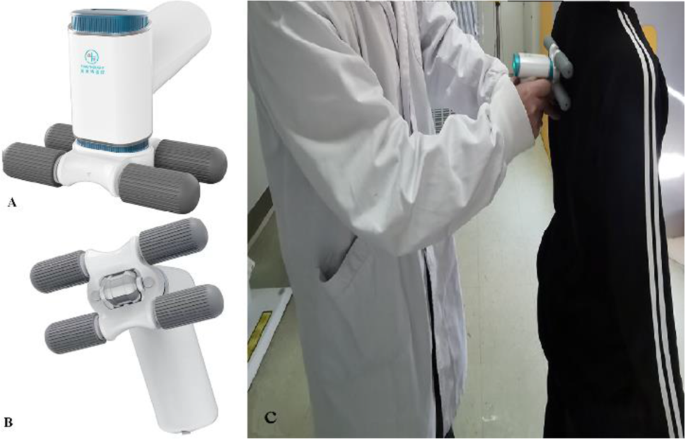
Fig. 3 Reconstrucción del modelo 3D de la columna vertebral y cálculo del ángulo de Cobb mediante 3D-SSS
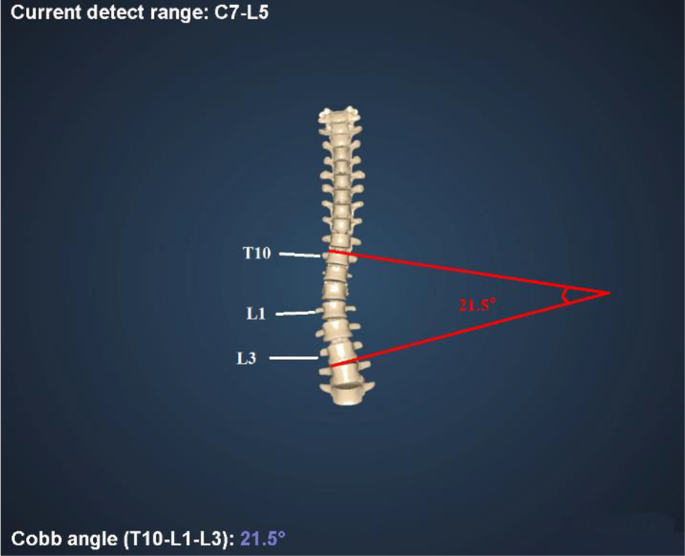
Cuadro 1 Datos demográficos de los 145 pacientes

Los sujetos fueron medidos de forma independiente por dos médicos: un radiólogo con seis años de experiencia en radiología musculoesquelética y un ortopedista con diez años de experiencia en cirugía de la columna vertebral. El ortopedista realizó dos mediciones en cada sujeto. Ambos médicos no conocían los resultados obtenidos con la EOS.
Análisis estadístico
Los parámetros cuantitativos se expresaron como media ± desviación estándar. Se calculó el coeficiente de correlación intraclase (CCI) para evaluar la variabilidad intraobservador e interobservador en las mediciones de la 3D-SSS. Los valores de ICC se interpretaron de la siguiente manera: >0,8 (excelente), 0,6-0,8 (bueno), 0,4-0,6 (moderado), 0,2-0,4 (leve) y < 0,2 (escasa fiabilidad). La concordancia, fiabilidad y correlación entre las mediciones de la EOS y la 3D-SSS se evaluaron mediante el análisis de Bland-Altman, el error cuadrático medio (RMSE), la CCI y el coeficiente de correlación de Pearson, respectivamente. El análisis estadístico se realizó mediante SPSS (versión 22.0, IBM, Armonk, NY, EE.UU.) y GraphPad Prism (versión 8.0.2, GraphPad Software, San Diego, CA, EE.UU.). El valor p < 0,05 se consideró estadísticamente significativo.
Resultados
En este estudio se incluyó a un total de 145 pacientes. Los datos demográficos de todos los pacientes se resumen en la Tabla 1. Entre ellos, 82 pacientes se sometieron a su primer examen radiográfico para la detección de escoliosis. La edad media de todos los pacientes fue de 19,9 ± 8,7 años (rango: 6∽39 años), con 88 pacientes de 18 años o menos (media: 13,7 ± 2,7 años, rango: 6∽18 años). Los CCI intraobservador e interobservador para el ángulo de Cobb derivado de la 3D-SSS fueron de 0,969 (IC 95%: 0,957-0,977) y 0,934 (IC 95%: 0,909-0,952), respectivamente, lo que indica una excelente reproducibilidad. El análisis de Bland-Altman (Fig. 4) reveló un sesgo de 0,1° para las mediciones intraobservador y de - 0,4° para las mediciones interobservador. Siete y cinco pacientes estaban fuera de los límites de concordancia (LOA) para las mediciones intraobservador (-4,2∽4,4°) e interobservador (-6,6∽5,8°), respectivamente. El RMSE para la concordancia intraobservador e interobservador fue de 2,1° y 3,1°, respectivamente.
El ángulo de Cobb medio medido mediante EOS y 3D-SSS fue de 13,7 ± 9,9° (intervalo: 0,5∽45,7°) y 12,5 ± 8,6° (intervalo: 0,4∽40°), respectivamente. La diferencia absoluta en el ángulo de Cobb entre la EOS y la 3D-SSS fue de 2,5 ± 2,9°. La fiabilidad de las mediciones del ángulo de Cobb entre la EOS y la 3D-SSS fue excelente [ICC = 0,921 (IC 95%: 0,893∽0,943)]. El análisis de Bland-Altman (Fig. 4) indicaron un sesgo de -1,171° entre EOS y 3D-SSS, con 10 pacientes fuera del LOA (-8,3∽6,0°). El RMSE entre EOS y 3D-SSS fue de 3,2°. El análisis de correlación de Pearson mostró una correlación muy estrecha entre las mediciones del ángulo de Cobb obtenidas mediante EOS y 3D-SSS (r= 0.931, P< 0.001). Además, las diferencias en las mediciones del ángulo de Cobb entre la EOS y la 3D-SSS aumentaron con ángulos de Cobb mayores, oscilando entre 1,5° y 12,7° a medida que el ángulo de Cobb aumentaba de 45° (Fig. 5).
El rendimiento diagnóstico de la 3D-SSS para la escoliosis (ángulo de Cobb ≥ 10°) se resume en la Tabla 2. La sensibilidad, especificidad, valor predictivo positivo y valor predictivo negativo de la 3D-SSS para el diagnóstico de escoliosis fueron 87,8%, 92,1%, 93,5% y 85,3%, respectivamente. Entre 63 pacientes con un ángulo de Cobb < 10°, sólo 5 fueron reclasificados a un ángulo de Cobb de 10∽25° por 3D-SSS. La curva receiver operating characteristic (ROC) demostró la gran capacidad predictiva de la 3D-SSS para la escoliosis, con un área bajo la curva (AUC) de 0,953 [IC 95%: 0,918∽0,988, P< 0,001] (Fig. 6). En 61 pacientes con un ángulo de Cobb de 10∽25°, 10 fueron reclasificados a un ángulo de Cobb 45° a un ángulo de Cobb de 25∽45°. Los resultados de reclasificación de la 3D-SSS se detallan en la Tabla 3.
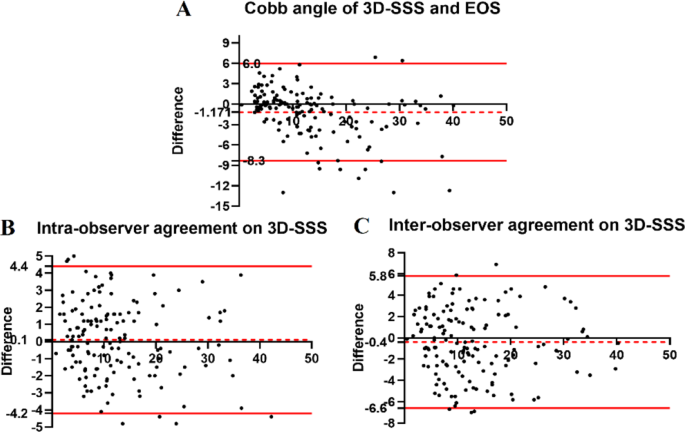
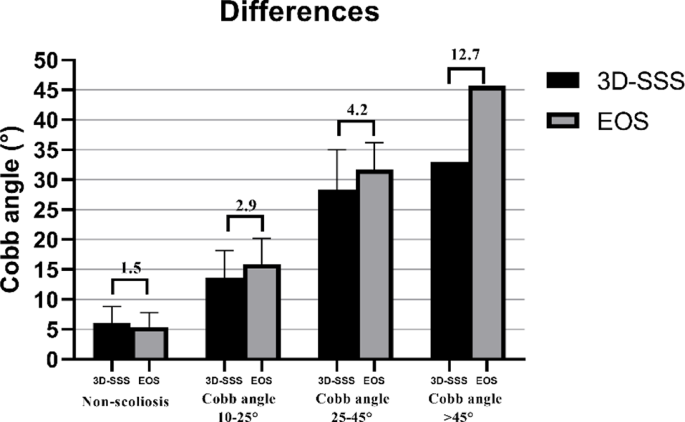
Cuadro 2 Capacidad diagnóstica de la escoliosis mediante 3D-SSS

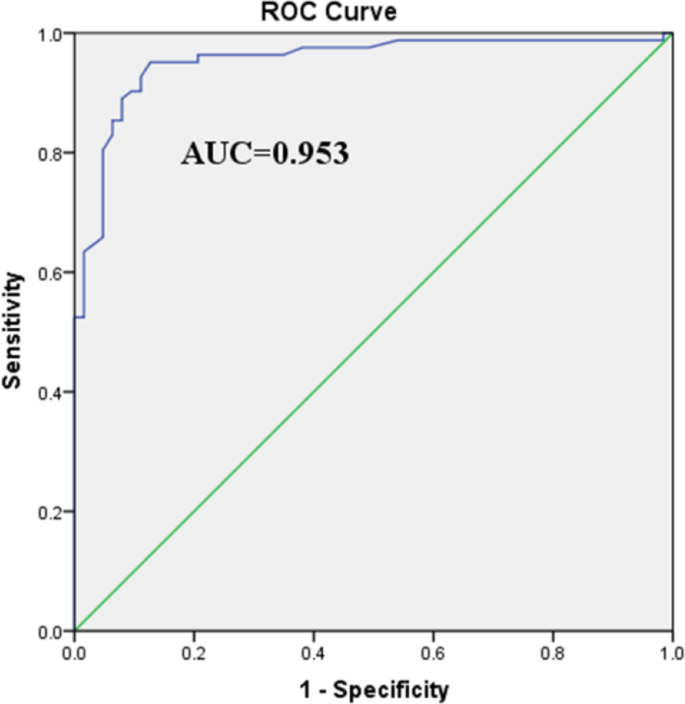
Cuadro 3 Reclasificación de la gravedad de la escoliosis mediante 3D-SSS

Debate
El presente estudio demostró que los ángulos de Cobb derivados de la reconstrucción automática de 3D-SSS estaban en buena concordancia con los obtenidos por EOS, con excelentes ICC intra e interobservador para 3D-SSS. Estos resultados indican que la 3D-SSS proporciona mediciones fiables y precisas para pacientes con escoliosis.
El ángulo de Cobb es un parámetro crítico para evaluar el AIS [17]. En la actualidad, la radiografía computarizada y la radiografía digital son los métodos más comunes para la evaluación cuantitativa del ángulo de Cobb mediante radiografías. El sistema EOS ha sido ampliamente adoptado desde su introducción, con su viabilidad y precisión en la evaluación de la AIS y la escoliosis del adulto confirmada por estudios previos [18,19,20,21,22]. Las investigaciones han demostrado un alto nivel de precisión en las mediciones del ángulo de Cobb obtenidas con EOS en comparación con la TC, tanto en estudios con maniquíes como con pacientes [18, 23, 24]. En consecuencia, la EOS se considera el patrón oro para evaluar la escoliosis mediante el ángulo de Cobb y la rotación vertebral axial (RVA). Sin embargo, los pacientes jóvenes con AIS sometidos a exposiciones repetidas a los rayos X se enfrentan a posibles daños debidos a la radiación [25].
Este estudio reveló que los ángulos de Cobb medidos por la novedosa 3D-SSS portátil y sin radiación son muy coherentes con los medidos por la EOS. Varios factores pueden explicar esta coherencia: (1) El método de recopilación de datos de 3D-SSS refleja el de EOS, capturando los parámetros frontales y laterales de la columna vertebral simultáneamente en condiciones de soporte de peso, lo que refleja fielmente la postura real del paciente en la vida cotidiana. (2) El sistema emplea un sensor MEMS de alta precisión que alcanza valores de exactitud teóricos de ≤ 1° en entornos libres de interferencias magnéticas. (3) El software de posprocesamiento ajusta automáticamente los datos recogidos a cada vértebra mediante algoritmos de fusión de sensores y control de filtros de ganancia, produciendo un modelo digital 3D de la columna vertebral de gran precisión. (4) El software de posprocesamiento traduce la técnica estándar de medición del ángulo de Cobb (determinación del ángulo entre los cuerpos vertebrales superior e inferior con inclinación máxima en un segmento de escoliosis) a un modelo matemático de vectores espaciales, automatizando los cálculos de los parámetros de la columna vertebral y reduciendo los errores manuales.
Estudios anteriores han investigado diversos métodos no radiográficos para la detección de la escoliosis y la evaluación del ángulo de Cobb. El escoliómetro combinado con la prueba de Adams es un método manual de uso común en la práctica clínica, pero carece de objetividad, precisión y repetibilidad [26]. Del mismo modo, los dispositivos electrónicos portátiles de detección de la escoliosis utilizan tecnología de detección electrónica de la gravedad en 3D para medir la rotación superficial del tronco y estimar la gravedad de la escoliosis, lo que puede producir resultados falsos positivos [27]. Además, Li et al. propusieron un método de ecografía 3D para evaluar el ángulo de Cobb utilizando tecnología ultrasónica para obtener imágenes de las apófisis espinosas dorsales del paciente y reconstruir la morfología de la columna vertebral. Su estudio informó de un coeficiente de correlación de > 0,75 entre el ángulo de Cobb y las apófisis espinosas dorsales, lo que sugiere que las mediciones basadas en ultrasonidos de la secuencia de las apófisis espinosas pueden lograr evaluaciones cuantitativas del ángulo de Cobb sin radiografías [28]. Sin embargo, un mal contacto sonda-piel o un gel de acoplamiento insuficiente pueden perjudicar la transmisión de la señal de ultrasonidos, afectando negativamente a la precisión de la medición [29].
Al igual que en el estudio de Li et al., este sistema utiliza la apófisis espinosa dorsal como punto de referencia anatómico. Sin embargo, a diferencia de los métodos basados en ultrasonidos que localizan la apófisis espinosa únicamente mediante imágenes, este sistema emplea un sensor combinado MEMS de alta precisión y tecnología de medición de trayectoria en espacio de contacto. El módulo de medición del ángulo del espacio de trayectoria y el módulo de medición de la distancia de trayectoria detectan con precisión la posición vectorial de cada apófisis espinosa en el espacio tridimensional. Además, los datos de exploración del terreno procedentes de la rueda de equilibrio optimizan la medición al tener en cuenta la inclinación y la torsión de la apófisis espinosa causadas por la tensión de los músculos de la espalda y el tejido adiposo. Por último, el mapeo digital de los datos, procesado mediante algoritmos de fusión y control de ganancia, genera un modelo de columna vertebral en 3D muy similar a la columna vertebral del paciente, lo que permite calcular y analizar el ángulo de Cobb [30, 31]. Este enfoque, que combina la localización vectorial 3D y la exploración topográfica de la apófisis espinosa dorsal, parece ser más eficaz que la ecografía por sí sola para localizar la apófisis espinosa coronal y evaluar el ángulo de Cobb.
Los resultados de este estudio tienen varias implicaciones clínicas. El AIS a menudo se presenta sin síntomas distintivos, lo que aumenta el riesgo de un diagnóstico erróneo o tardío. La detección precoz de la escoliosis mediante el cribado por imagen permite una intervención oportuna, como el uso de ortesis o procedimientos quirúrgicos [25]. Aunque el EOS es preciso y fiable para la evaluación de la escoliosis, su uso está limitado a salas de examen especializadas y requiere la operación por parte de radiógrafos formados. El EOS también tiene inconvenientes, como los elevados costes iniciales, los tiempos de examen relativamente largos y la exposición a la radiación, lo que lo hace inadecuado para el cribado a gran escala del SIA [11, 32]. En cambio, el dispositivo utilizado en este estudio es portátil, no emite radiación y es rentable. El coste de instalación inicial de un sistema EOS es de aproximadamente $509.480, con un coste por exploración de $11,58 [32]. En comparación, el coste de adquisición de la 3D-SSS es de aproximadamente $41.430, y el coste por paciente es de aproximadamente $4,14, ambos significativamente inferiores a los de la EOS. Además, este estudio encontró una fuerte consistencia y correlación entre las mediciones obtenidas de 3D-SSS y EOS, junto con la alta precisión diagnóstica de 3D-SSS, por lo que es adecuado para la detección de AIS en comunidades, escuelas y entornos de atención primaria. La detección precoz de la escoliosis mediante herramientas como los escoliógrafos permite intervenciones no quirúrgicas oportunas, como el uso de corsés, que pueden ralentizar la progresión [33, 34], y la cirugía en las fases adecuadas para evitar las complicaciones asociadas a la escoliosis avanzada [35]. El novedoso dispositivo 3D-SSS también tiene potencial para monitorizar la progresión de la enfermedad y los resultados del tratamiento, con implicaciones pronósticas. Además, el software del dispositivo proporciona resultados en 10 s, lo que agiliza el diagnóstico clínico y la gestión. Por lo tanto, el dispositivo sin radiación ofrece una herramienta práctica y eficaz para la evaluación de la escoliosis.
Sin embargo, el estudio tiene algunas limitaciones: (1) El tamaño de la muestra fue pequeño, sobre todo en el caso de los pacientes con deformidades graves del ángulo de Cobb. Los estudios futuros deberían incluir más pacientes con deformidades graves. (2) No se evaluó la influencia de la 3D-SSS en las estrategias de tratamiento clínico. Por ejemplo, los pacientes a los que se recomienda una intervención quirúrgica basada en la EOS podrían ser tratados con ortesis según la 3D-SSS, lo que podría afectar a la corrección de la curva. Por el contrario, los pacientes a los que se recomienda observación según el EOS podrían recibir un tratamiento excesivo con corsé. Se necesitan más estudios para evaluar las implicaciones clínicas. (3) El estudio carecía de datos de seguimiento, lo que impedía realizar comparaciones de un mismo paciente a lo largo del tiempo. (4) Sólo dos médicos (un radiólogo y un traumatólogo) realizaron los exámenes. En futuros estudios deberían participar varios operadores para evaluar la coherencia del sistema. (5) Aunque se obtuvieron resultados prometedores con pacientes que llevaban ropa fina, futuros estudios deberían investigar si las mediciones mejoran con el contacto directo con la piel.
Conclusiones
En conclusión, el 3D-SSS proporciona evaluaciones de escoliosis precisas y reproducibles en adolescentes y adultos jóvenes, con mediciones muy coherentes con la EOS. Este sistema puede complementar la EOS y ayudar a los médicos a diagnosticar la escoliosis de forma rápida y precisa. Sin embargo, se necesita una mayor validación para los pacientes con ángulos de Cobb graves.
Abreviaturas
3D-SSS: Sistema de detección tridimensional de la columna vertebral
3D: Tridimensional
AIS: Escoliosis idiopática del adolescente
MEMS: Sistema microelectromecánico
LED: Diodo emisor de luz
MCU: Unidad de microcontrolador
ICC: Coeficiente de correlación intraclase
RMSE: Error cuadrático medio
LOA: Límites del acuerdo
ROC: Característica operativa del receptor
AUC: Área bajo la curva
CI: Intervalo de confianza
IMC: Índice de masa corporal
Agradecimientos
No se aplica.
Contribuciones de los autores
Todos los autores leyeron y aprobaron el manuscrito final. Hui Wang: redacción del trabajo Fengyun Zhu: adquisición, análisis e interpretación de los datos Qiyuan Bao: adquisición, análisis e interpretación de los datos Yong Lu: adquisición, análisis e interpretación de los datos Fuhua Yan: concepción y diseño del trabajo Lianjun Du: revisión sustancial del trabajo Le Qin: concepción y diseño del trabajo, revisión sustancial del trabajo.
Información sobre el autor
Autores y afiliaciones
- Departamento de Radiología, Hospital Ruijin, Facultad de Medicina de la Universidad Jiao Tong de Shanghai, No. 197 Ruijin 2nd Rd, Shanghai, 200025, ChinaHui Wang, Yunfeng Zhu, Yong Lu, Fuhua Yan, Lianjun Du & Le Qin
- Departamento de Ortopedia, Hospital Ruijin, Facultad de Medicina de la Universidad Jiao Tong de Shanghai, No. 197 Ruijin 2nd Rd, Shanghai, 200025, ChinaQiyuan Bao
Financiación
Este estudio ha sido financiado por el Programa Nacional Clave de I+D de China (2023YFC2410704) y la Fundación Nacional de Ciencias Naturales de China (82171891).
Disponibilidad de datos
Los conjuntos de datos utilizados y analizados en el presente estudio pueden solicitarse al autor correspondiente.
Declaraciones
Aprobación ética y consentimiento para participar
Se obtuvo la aprobación ética y el consentimiento del Comité de Ética del Hospital Ruijin de la Facultad de Medicina de la Universidad JiaoTong de Shanghai. El número de referencia es el nº (2022)(273). Sólo si el estudio se realiza en seres humanos: En este estudio se obtuvo el consentimiento informado por escrito de todos los sujetos (pacientes) o de sus tutores legales.
Consentimiento para la publicación
No se aplica.
Intereses contrapuestos
Los autores declaran no tener intereses contrapuestos.
Recibido: 19 de marzo de 2024 / Aceptado: 11 de febrero de 2025
Publicado en línea: 26 febrero 2025
Referencias
- 1. Marya S, Tambe AD, Millner PA, Tsirikos AI. Adolescent idiopathic scoliosis: a review of aetiological theories of a multifactorial disease. Bone Joint J. 2022;104:915-21.
- 2. Peng Y, W ang SR, Qiu GX, Zhang JG, Zhuang QY. Research progress on the etiology and pathogenesis of adolescent idiopathic scoliosis. Chin Med J. 2020;133:483-93.
- 3. Guo X, Chau WW, Hui-Chan CW, Cheung CS, Tsang WW, Cheng JC. Balance control in adolescents with idiopathic scoliosis and disturbed somatosensory function. Spine. 2006;31(14):e437-40.
- 4. Song XX, Jin LY, Li XF, Qian L, Shen HX, Liu ZD, et al. Efectos del bajo estado mineral óseo en las características biomecánicas en la deformidad espinal escoliótica idiopática. World Neurosurg. 2018;110:e321-9.
- 5. Konieczny MR, Senyurt H, Krauspe R. Epidemiology of adolescent idiopathic scoliosis. J Child Orthop. 2013;7:3-9.
- 6. Aebi M. La escoliosis del adulto. Eur Spine J. 2005;14:925-48.
- 7. Cheng JC, Castelein RM, Chu WC, et al. Adolescent idiopathic scoliosis. Nat Rev Dis Primers. 2015;1:15030.
- 8. Weinstein SL, Dolan LA, Spratt KF, Peterson KK, Spoonamore MJ, Ponseti IV. Health and function of patients with untreated idiopathic scoliosis. A 50-year natural history study. JAMA. 2003;289:559-67.
- 9. Dubousset J, Charpak G, Skalli W, Deguise J, Kalifa G. EOS: a new imaging system with low dose radiation in standing position for spine and bone & joint disorders. J Musculoskelet Res. 2010;13:1-12.
- 10. Harada GK, Siyaji ZK, Younis S, Louie PK, Samartzis D, An HS. Imágenes en cirugía de columna: conceptos actuales y direcciones futuras. Spine Surg Relat Res. 2019;4:99-110.
- 11. Garg B, Mehta N, Bansal T, Malhotra R. EOS imaging: concept and current applications in spinal disorders. J Clin Orthop Trauma. 2020;11:786-93.
- 12. Luo TD, Stans AA, Schueler BA, Larson AN. Cumulative radiation exposure with EOS imaging compared with standard spine radiographs. Spine Deform. 2015;3:144-50.
- 13. Melhem E, Assi A, El Rachkidi R, Ghanem I. EOS biplanar X-ray imaging: concept, developments, benefits, and limitations. J Child Orthop. 2016;10:1-14.
- 14. Algamili AS, Khir MHM, Dennis JO, et al. A review of actuation and sensing mechanisms in MEMS-based sensor devices. Nanoscale Res Lett. 2021;16:16.
- 15. Sabatini AM. Estimating three-dimensional orientation of human body parts by inertial/magnetic sensing. Sensors. 2011;11:1489-525.
- 16. Carmi A, Oshman Y. Adaptive particle filtering for spacecraft attitude estimation from vector observations. J Guid Control Dynam. 2009;32:232-41.
- 17. Rigo M. Evaluación del paciente en la escoliosis idiopática: valoración radiográfica, deformidad del tronco y asimetría de la espalda. Physiother Theory Pract. 2011;27:7-25.
- 18. Al-Aubaidi Z, Lebel D, Oudjhane K, Zeller R. Three-dimensional imaging of the spine using the EOS system: is it reliable? A comparative study using computed tomography imaging. J Pediatr Orthop B. 2013;22:409-12.
- 19. Rehm J, Germann T, Akbar M, et al. 3D-modeling of the spine using EOS imaging system: inter-reader reproducibility and reliability. PLoS ONE. 2017;12:1-13.
- 20. Okamoto M, Jabour F, Sakai K, Hatsushikano S, Le Huec JC, Hasegawa K. Sagittal balance measures are more reproducible when measured in 3D vs in 2D using full-body EOS images. Eur Radiol. 2018;28:4570-7.
- 21. Chan AC, Morrison DG, Nguyen DV, Hill DL, Parent E, Lou EH. Intra- and interobserver reliability of the Cobb angle-vertebral rotation angle-spinous process angle for adolescent idiopathic scoliosis. Spine Deform. 2014;2:168-75.
- 22. Kim SB, Heo YM, Hwang CM, et al. Fiabilidad del sistema de imágenes EOS para la evaluación de la alineación de la columna vertebral y la pelvis en el plano sagital. Clin Orthop Surg. 2018;10:500-7.
- 23. Glaser DA, Doan J, Newton PO. Comparison of 3-dimensional spinal reconstruction accuracy: biplanar radiographs with EOS versus computed tomography. Spine (Phila Pa 1976). 2012;37:1391-7.
- 24. Carreau JH, Bastrom T, Petcharaporn M, et al. Computer-generated, three-dimensional spine model from biplanar radiographs: a validity study in idiopathic scoliosis curves greater than 50 degrees. Spine Deform. 2014;2:81-8.
- 25. Rigo MD, Villagrasa M, Gallo D. A specific scoliosis classification correlating with brace treatment: description and reliability. Scoliosis. 2010;5:1-11.
- 26. Fong DY, Lee CF, Cheung KM, et al. A meta-analysis of the clinical effectiveness of school scoliosis screening. Spine (Phila Pa 1976). 2010;35:1061-71.
- 27. Li C, Zhang B, Liu L, et al. Design, reliability, and validity of a portable electronic device based on ergonomics for early screening of adolescent scoliosis. J Orthop Translat. 2021;28:83-9.
- 28. Li M, Cheng J, Ying M, Ng B, Lam TP, Wong MS. A preliminary study of estimation of Cobb's angle from the spinous process angle using a clinical ultrasound method. Spine Deform. 2015;3:476-82.
- 29. Lai KK, Lee TT, Lee MK, Hui JC, Zheng YP. Validation of scolioscan air-portable radiation-free three-dimensional ultrasound imaging assessment system for scoliosis. Sensors(Basel). 2021;21:2858-75.
- 30. Giansanti D, Macellari V, Maccioni G, Cappozzo A. ¿Es factible reconstruir la posición y orientación tridimensional de segmentos corporales utilizando datos acelerométricos? IEEE Trans Biomed Eng. 2003;50:476-83.
- 31. Cortell-Tormo JM, Garcia-Jaen M, Ruiz-Fernandez D, Fuster-Lloret V. Lumbatex: a wearable monitoring system based on inertial sensors to measure and control the lumbar spine motion. IEEE Trans Neural Syst Rehabil Eng. 2019;27:1644-53.
- 32. Faria R, McKenna C, Wade R, Yang H, Woolacott N, Sculpher M. The EOS 2D/3D X-ray imaging system: a cost-effectiveness analysis quantifying the health benefits from reduced radiation exposure. Eur J Radiol. 2013;82:342-9.
- 33. Diebo BG, Segreto FA, Solow M, et al. Adolescent idiopathic scoliosis care in an underserved inner-city population: screening, bracing, and patient- and parent-reported outcomes. Spine Deform. 2019;7:559-64.
- 34. Grossman DC, Curry SJ, Owens DK, et al. Screening for adolescent idiopathic scoliosis: US preventive services task force recommendation statement. JAMA. 2018;319:165-72.
- 35. Adobor RD, Rimeslatten S, Steen H, Brox JI. School screening and point prevalence of adolescent idiopathic scoliosis in 4000 Norwegian children aged 12 years. Scoliosis. 2011;6:1-7.
Nota del editor
Springer Nature se mantiene neutral con respecto a las reclamaciones jurisdiccionales en los mapas publicados y las afiliaciones institucionales.
Acceso libre Este artículo está bajo una Licencia Creative Commons Atribución-NoComercial-SinObraDerivada 4.0 Internacional, que permite cualquier uso no comercial, compartir, distribuir y reproducir en cualquier medio o formato, siempre que se cite debidamente al autor o autores originales y la fuente, se proporcione un enlace a la licencia Creative Commons y se indique si se ha modificado el material autorizado. En virtud de esta licencia, no tiene permiso para compartir material adaptado derivado de este artículo o de partes del mismo. Las imágenes u otro material de terceros en este artículo están incluidos en la licencia Creative Commons del artículo, a menos que se indique lo contrario en una línea de crédito al material. Si el material no está incluido en la licencia Creative Commons del artículo y su uso previsto no está permitido por la normativa legal o excede el uso permitido, deberá obtener permiso directamente del titular de los derechos de autor. Para ver una copia de esta licencia, visite http://creativecommons.org/licenses/by-nc-nd/4.0/.